Home>Science>The Surprising Difference Between Longitudinal And Transverse Waves
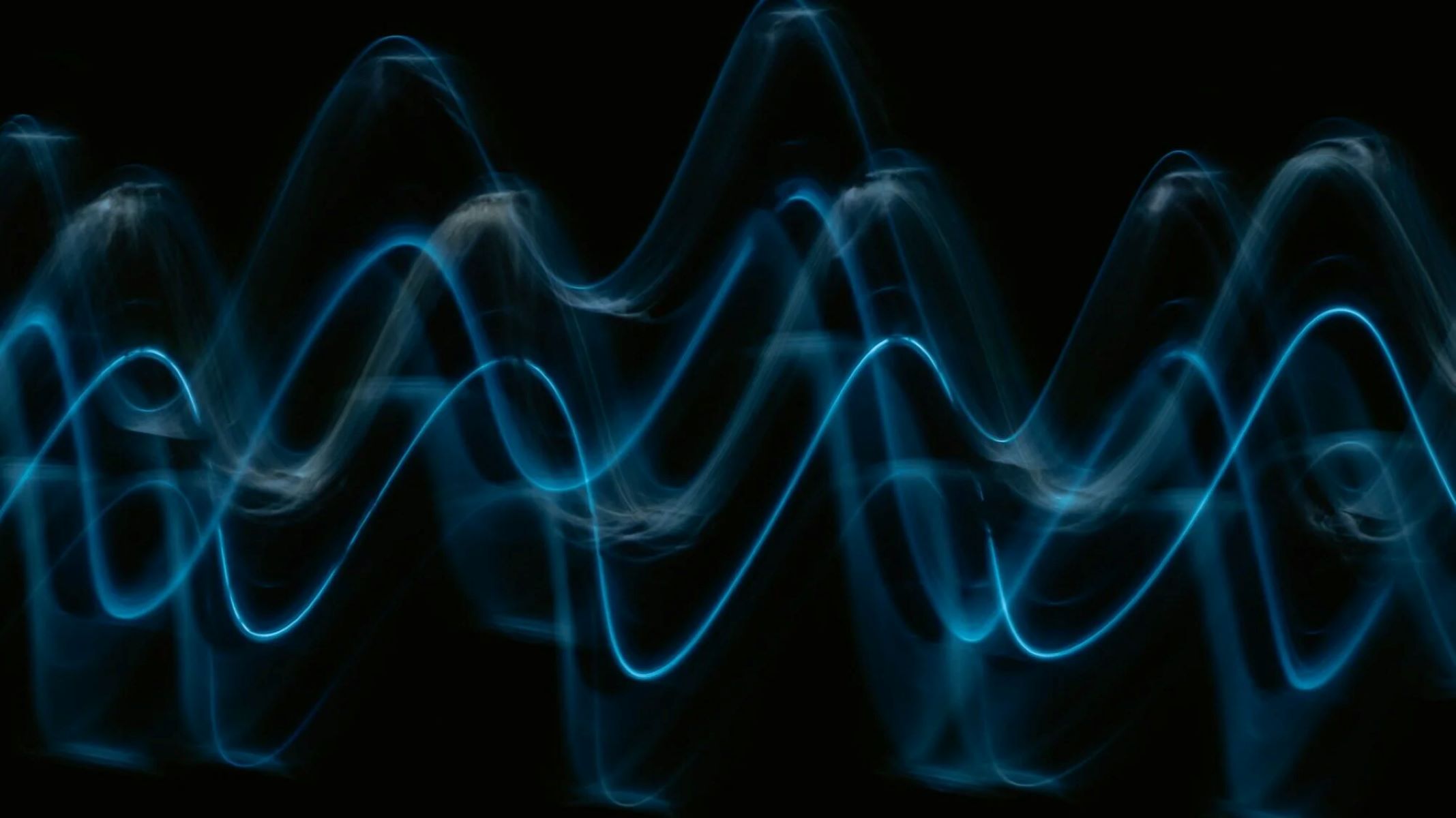
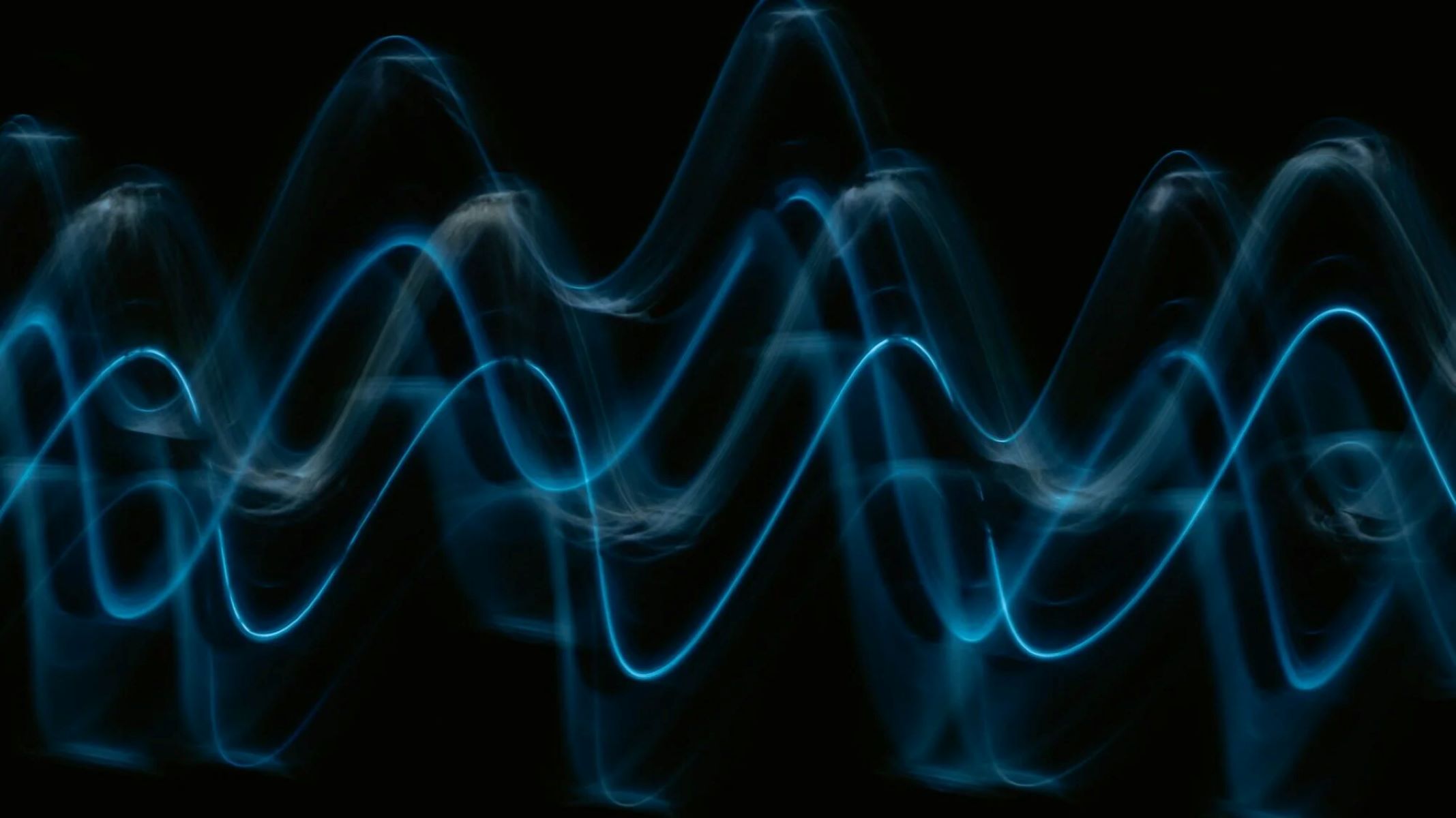
Science
The Surprising Difference Between Longitudinal And Transverse Waves
Published: January 27, 2024
Discover the key disparities between longitudinal and transverse waves in science. Learn how these wave types differ and their unique characteristics.
(Many of the links in this article redirect to a specific reviewed product. Your purchase of these products through affiliate links helps to generate commission for Regretless.com, at no extra cost. Learn more)
Table of Contents
Introduction
Waves are an integral part of our daily lives, from the gentle ripples on a serene lake to the powerful seismic waves that shape the Earth's crust. They are ubiquitous in the natural world and play a crucial role in various scientific and technological applications. One of the fundamental distinctions in the realm of waves lies in the differentiation between longitudinal and transverse waves. Understanding the unique characteristics and behaviors of these wave types is essential for comprehending a wide array of phenomena, from sound propagation to the transmission of seismic energy.
In this article, we will delve into the intriguing realm of longitudinal and transverse waves, unraveling their distinct properties and shedding light on their diverse applications. By exploring the underlying principles that govern these wave types, we will gain a deeper appreciation for the intricate nature of wave propagation and its profound impact on the world around us. So, let's embark on a captivating journey through the fascinating domain of longitudinal and transverse waves, where we will uncover the surprising differences that set them apart and explore the remarkable ways in which they shape our understanding of the physical universe.
Understanding Longitudinal Waves
Longitudinal waves are a captivating manifestation of wave behavior, characterized by the unique manner in which they propagate energy through a medium. Unlike transverse waves, which exhibit perpendicular oscillations relative to the direction of wave travel, longitudinal waves feature oscillations that align parallel to the direction of propagation. This distinctive attribute gives rise to a fascinating mode of wave motion, wherein compressions and rarefactions travel through the medium, imparting energy and transmitting disturbances in a remarkable fashion.
At the heart of longitudinal waves lies the concept of compression and rarefaction. As the wave propagates through a medium, it induces regions of high pressure, known as compressions, followed by areas of low pressure, referred to as rarefactions. This alternating pattern of compression and rarefaction serves as the hallmark of longitudinal wave behavior, shaping the dynamic interplay of energy transfer within the medium.
A classic example of longitudinal waves in action is the transmission of sound through air. When a sound wave is generated, such as the melodic strumming of a guitar string, it sets off a series of compressions and rarefactions in the air molecules. These oscillations travel longitudinally, causing the molecules to crowd together in regions of compression and spread apart in regions of rarefaction. This intricate dance of molecular motion carries the acoustic energy of the sound wave, allowing it to propagate through the air and reach our ears, where it is perceived as the delightful harmony of musical notes.
In addition to sound waves, longitudinal wave phenomena are also prevalent in seismic waves that traverse the Earth's interior. When an earthquake occurs, the release of seismic energy gives rise to longitudinal waves, known as primary or P-waves, which propagate through the Earth's crust. These waves exhibit a compressional nature, causing the ground to undergo alternating periods of compression and expansion as the seismic energy courses through the planet's subsurface layers.
The captivating nature of longitudinal waves extends beyond the realms of sound and seismic activity, permeating various branches of science and engineering. By unraveling the intricate dynamics of longitudinal wave propagation, researchers and innovators continue to harness the potential of these waves in diverse applications, from medical imaging techniques to industrial non-destructive testing methods.
As we delve deeper into the realm of longitudinal waves, we uncover a rich tapestry of scientific principles and practical implications, underscoring the profound significance of these waves in shaping our understanding of the physical world. Their unique mode of energy transmission and their pervasive presence in natural phenomena serve as a testament to the captivating allure of longitudinal waves, inviting us to explore their intricacies and unravel the mysteries of wave propagation in all its wondrous complexity.
Understanding Transverse Waves
Transverse waves are a captivating manifestation of wave behavior, characterized by the unique manner in which they propagate energy through a medium. Unlike longitudinal waves, which exhibit oscillations parallel to the direction of wave travel, transverse waves feature oscillations that align perpendicular to the direction of propagation. This distinctive attribute gives rise to a fascinating mode of wave motion, wherein the medium undergoes periodic displacement in a direction perpendicular to the wave's path.
At the heart of transverse waves lies the concept of crest and trough. As the wave propagates through a medium, it induces regions of upward displacement, known as crests, followed by areas of downward displacement, referred to as troughs. This alternating pattern of crest and trough serves as the hallmark of transverse wave behavior, shaping the dynamic interplay of energy transfer within the medium.
A classic example of transverse waves in action is the propagation of electromagnetic radiation, including visible light and radio waves. When electromagnetic waves are generated, such as the radiant glow of a light bulb or the transmission of radio signals, they exhibit transverse wave behavior. The electric and magnetic fields oscillate perpendicular to the direction of wave travel, creating a captivating dance of energy propagation that underpins the wondrous phenomena of light and wireless communication.
In addition to electromagnetic waves, transverse wave phenomena are also prevalent in the realm of mechanical vibrations, such as the undulating motion of a taut guitar string or the rippling oscillations of a drum membrane. These mechanical waves manifest transverse behavior, as the particles of the medium undergo perpendicular displacement relative to the direction of wave propagation, giving rise to a rich tapestry of harmonic motion and resonant frequencies.
The captivating nature of transverse waves extends beyond the realms of electromagnetic radiation and mechanical vibrations, permeating various branches of science and engineering. By unraveling the intricate dynamics of transverse wave propagation, researchers and innovators continue to harness the potential of these waves in diverse applications, from optical communication technologies to the study of seismic wave polarization in geophysics.
As we delve deeper into the realm of transverse waves, we uncover a rich tapestry of scientific principles and practical implications, underscoring the profound significance of these waves in shaping our understanding of the physical world. Their unique mode of energy transmission and their pervasive presence in natural and engineered phenomena serve as a testament to the captivating allure of transverse waves, inviting us to explore their intricacies and unravel the mysteries of wave propagation in all its wondrous complexity.
Key Differences Between Longitudinal and Transverse Waves
The distinction between longitudinal and transverse waves lies at the heart of wave dynamics, encompassing a myriad of contrasting features that shape their behavior and applications. These fundamental differences serve as the cornerstone for understanding the diverse manifestations of wave phenomena and their profound impact on the natural world. Let's unravel the key disparities between these two wave types, delving into their unique characteristics and the captivating ways in which they propagate energy through various mediums.
-
Direction of Particle Displacement: One of the primary disparities between longitudinal and transverse waves lies in the direction of particle displacement within the medium. In longitudinal waves, the particles oscillate parallel to the direction of wave propagation, leading to compressions and rarefactions that traverse the medium in a linear fashion. Conversely, transverse waves induce particle displacement perpendicular to the direction of wave travel, giving rise to crests and troughs that propagate through the medium in a captivating oscillatory motion.
-
Nature of Oscillations: Longitudinal waves exhibit a compressional nature, wherein the medium experiences alternating regions of compression and rarefaction as the wave energy propagates. This compressional behavior results from the parallel oscillations of particles, leading to a dynamic interplay of high and low-pressure zones within the medium. In contrast, transverse waves manifest a distinctive pattern of crest and trough oscillations, characterized by perpendicular displacement of particles that engenders a captivating undulating motion within the medium.
-
Propagation Characteristics: Longitudinal waves propagate energy through the medium by transmitting compressions and rarefactions in a linear fashion, allowing the wave to traverse the medium with remarkable efficiency. This mode of propagation enables longitudinal waves to transmit energy over long distances, making them well-suited for phenomena such as sound transmission and seismic wave propagation. On the other hand, transverse waves propagate energy through the medium by inducing crests and troughs that traverse perpendicular to the direction of wave travel, leading to a captivating mode of energy transmission that underpins phenomena such as electromagnetic radiation and mechanical vibrations.
-
Applications and Phenomena: The distinct characteristics of longitudinal and transverse waves give rise to diverse applications and phenomena across various scientific and technological domains. Longitudinal waves find widespread utility in fields such as acoustics, seismology, and medical imaging, where their compressional nature and efficient propagation enable a range of practical applications. Transverse waves, on the other hand, are prevalent in phenomena such as electromagnetic radiation, mechanical vibrations, and optical communication, showcasing their pivotal role in enabling technologies and natural phenomena that shape our modern world.
By unraveling these key disparities, we gain a deeper appreciation for the captivating differences between longitudinal and transverse waves, shedding light on their unique properties and the remarkable ways in which they influence our understanding of wave dynamics and their applications in the natural and engineered world.
Applications of Longitudinal and Transverse Waves
The distinctive characteristics of longitudinal and transverse waves pave the way for a diverse array of applications across numerous scientific and technological domains. These applications leverage the unique propagation mechanisms and behaviors of longitudinal and transverse waves to enable a wide range of phenomena and innovations that shape our modern world.
Applications of Longitudinal Waves
Acoustics and Sound Engineering:
Longitudinal waves play a pivotal role in the realm of acoustics and sound engineering, where their compressional nature and efficient propagation through mediums such as air, water, and solids enable the transmission of sound. From the design of concert hall acoustics to the development of high-fidelity audio systems, the understanding of longitudinal wave behavior forms the bedrock of immersive auditory experiences and cutting-edge sound technologies.
Seismic Wave Analysis:
In seismology, the study of longitudinal waves, also known as primary or P-waves, provides invaluable insights into the composition and dynamics of the Earth's interior. By analyzing the propagation of longitudinal waves through the planet's subsurface layers, geophysicists can map seismic activity, detect underground structures, and unravel the mysteries of the Earth's geological processes, contributing to advancements in earthquake monitoring and geological exploration.
Medical Imaging Techniques:
The compressional nature of longitudinal waves finds remarkable utility in medical imaging techniques such as ultrasound, where the propagation of acoustic waves through biological tissues enables non-invasive visualization of internal organs and structures. By harnessing the principles of longitudinal wave propagation, healthcare professionals can obtain detailed diagnostic images, perform prenatal screenings, and guide minimally invasive medical procedures, revolutionizing the field of diagnostic medicine.
Applications of Transverse Waves
Electromagnetic Radiation and Communication Technologies:
Transverse waves, in the form of electromagnetic radiation, underpin a myriad of communication technologies, including radio transmission, television broadcasting, and wireless networking. The oscillatory propagation of transverse waves enables the transmission of information through the modulation of electromagnetic fields, shaping the landscape of modern telecommunications and fostering global connectivity.
Optical Technologies and Imaging Systems:
In the realm of optics, transverse waves manifest in the propagation of light and the functioning of optical imaging systems. From the development of advanced microscopy techniques to the design of high-resolution cameras and imaging devices, the unique behavior of transverse waves facilitates innovations in visual technologies, scientific imaging, and the exploration of the cosmos through telescopic observations.
Material Analysis and Non-Destructive Testing:
Transverse waves find practical applications in material analysis and non-destructive testing methods, where their oscillatory nature enables the detection of internal flaws, structural integrity assessments, and characterization of material properties. Through techniques such as ultrasonic testing and eddy current inspection, engineers and researchers leverage the behavior of transverse waves to ensure the safety and reliability of critical infrastructure, industrial components, and aerospace systems.
By harnessing the distinct properties of longitudinal and transverse waves, scientists, engineers, and innovators continue to unlock the potential of these wave types in a myriad of applications, from enhancing our understanding of the natural world to driving technological advancements that enrich our lives and expand the frontiers of human knowledge.
Conclusion
In conclusion, the exploration of longitudinal and transverse waves unveils a captivating tapestry of scientific principles, practical applications, and profound implications for our understanding of the physical world. The distinctive characteristics of these wave types, from the compressional nature of longitudinal waves to the oscillatory behavior of transverse waves, underpin a myriad of natural phenomena and technological innovations that permeate our daily lives.
Longitudinal waves, with their parallel oscillations and efficient propagation of compressions and rarefactions, find widespread utility in fields such as acoustics, seismology, and medical imaging. From the transmission of sound in the air to the analysis of seismic activity within the Earth's interior, longitudinal waves play a pivotal role in shaping our understanding of auditory experiences, geological processes, and diagnostic medicine.
On the other hand, transverse waves, with their perpendicular oscillations and captivating propagation of crests and troughs, underpin a diverse array of applications in electromagnetic radiation, optical technologies, and material analysis. From the transmission of radio signals to the exploration of the cosmos through telescopic observations, transverse waves drive advancements in communication technologies, visual imaging, and non-destructive testing methods.
The key disparities between longitudinal and transverse waves, encompassing the direction of particle displacement, nature of oscillations, propagation characteristics, and applications, highlight the remarkable diversity and impact of these wave types on our modern world. By unraveling these disparities, we gain a deeper appreciation for the captivating differences between longitudinal and transverse waves, shedding light on their unique properties and the remarkable ways in which they influence our understanding of wave dynamics and their applications in the natural and engineered world.
As we reflect on the profound significance of longitudinal and transverse waves, we are reminded of the intricate interplay between scientific inquiry, technological innovation, and the awe-inspiring phenomena that shape our existence. The exploration of these wave types serves as a testament to the boundless potential of human curiosity and ingenuity, inviting us to delve deeper into the mysteries of wave propagation and embrace the transformative power of scientific exploration.
In essence, the study of longitudinal and transverse waves transcends the confines of scientific disciplines, offering a glimpse into the wondrous complexities of the universe and inspiring the relentless pursuit of knowledge and discovery. It is through this pursuit that we continue to unravel the mysteries of wave phenomena, harness their potential in diverse applications, and embark on a journey of exploration that transcends the boundaries of what we perceive as possible.